
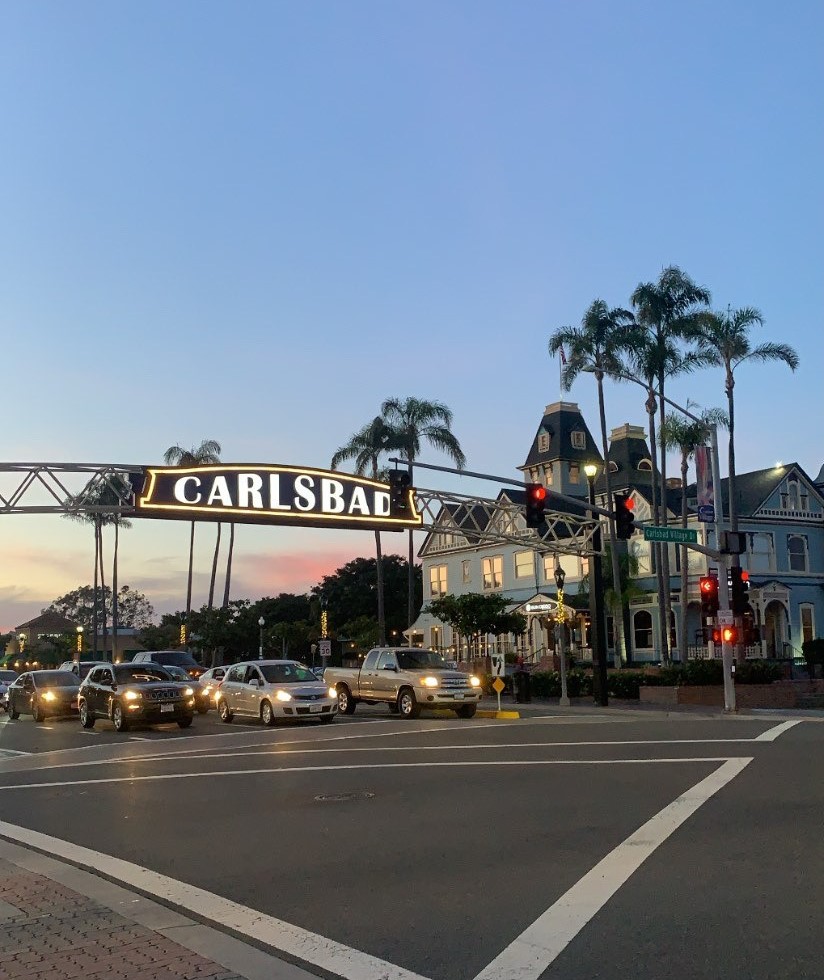

Individual ES colonies were removed by dislodging the micropallet with a single 5-ns laser pulse focused to a micron-sized spot at the interface of the micropallet’s base and glass substrate ( 14). A two-step procedure was used to release and collect target colonies. Colonies composed of undifferentiated cells could easily be identified by inspecting the morphology of the cells and colonies under a microscope. When the cells were plated at densities yielding 1 or 0 cells per micropallet and then cultured, clonal colonies were generated on the pallets initially receiving a single cell ( 16). In this work, strain 129 murine ES cells were seeded on 1002F micropallets by allowing them to settle onto the array from a suspension. By virtue of the small dimensions and ease of handling, cells can be cultured and manipulated using small volumes of media, an advantage when expensive growth factors such as LIF are required. Typically, a 1.6 × 1.6 cm array can contain more than 10 5 individual micropallets depending on their dimensions. The micropallets are formed from a biocompatible photoresist ( e.g., SU-8, 1002F) which is photolithographically defined on a standard glass microscope slide to create the array ( 15). Each micropallet with its attached cell/colony can be released from the array on an individual basis using a pulsed laser and then collected in order to sort and expand cells/colonies of interest. This technology is comprised of an array formed from transparent pedestals of micron-size dimensions, termed micropallets, on which cells are cultured. Recently, a new cell sorting strategy using lab-on-a-chip techniques has been developed that promises a number of advantages for ES cell sorting and cloning ( 13- 17). In response to these needs, there has been an effort to develop microengineered devices for stem cell applications ( 7- 12). Correspondingly, new technologies to support generation of these cell lines are in high demand. Implementation of new techniques can be expected to enhance the speed and decrease the costs compared with conventional methods for generating stable gene-targeted clones. It can be expected that the production of ES cell lines will be a long-term practice for modeling human disease, pharmaceutical testing, and in creating GEMs in various mouse strains that, while less robust than the 129 and C57BL/6 strains used for large-scale projects, are preferred in specific research areas ( 6).
#Carlsbad hourworld manual#
These efforts have utilized robotics to automate many of the steps in GEM production, yet choosing and isolating colonies by manual colony picking remains a bottleneck in this process ( 3, 6).

Large consortia have been organized to produce mouse models on a massive scale ( 6). Samples from each colony are then prepared for genetic analysis ( e.g., PCR) ( 5). Each prospective colony is manually picked with a pipette, disaggregated, and further expanded. Colonies composed of undifferentiated cells are identified microscopically by their cellular and colony morphology ( 4). After 2 weeks of cell expansion, clonal colonies of adequate size for manual picking are usually present. While elimination of the feeder layer reduces complexity, purified LIF is an expensive reagent that must be used in significant quantities for the lengthy culture and volumes of media conventionally required to establish cell lines. Typically, the media must include leukemia inhibitory factor (LIF), a cytokine necessary for maintaining the ES cells in an undifferentiated state in the absence of a feeder cell layer ( 3). The remaining cells are then grown for 2 weeks in 10-cm tissue culture dishes in media containing selective antibiotics.

In conventional methods, typically 20 × 10 6 cells are electroporated in the presence of the transfection vector with only 10-50% of cells surviving this transfection step. The selection and isolation of clonal ES colonies for generating cell lines and for producing GEMs is a time-intensive and laborious process. There are now widespread efforts underway to produce genetically engineered mice (GEMs) representing gene “knock outs” for each gene in the mouse genome and for “knock-in” of humanized genes in the modeling of human diseases ( 1, 2). The ability to genetically modify murine embryonic stem (ES) cells through gene targeting and homologous recombination has had a tremendous impact on biological and biomedical research ( 1).
